Welcome to the Decarb Guidebook
Approach
Benchmarking
Building Codes & Design Standards
State & Local Regulations
Technologies: Load Reduction
Technologies: Dehumidification
Technologies: If you must have a gas-fired boiler
Technologies: Central Plant
Technologies: Domestic Hot Water
Technologies: Steam
Technologies: Load Shifting and Energy Storage
Technologies: Emerging Technologies
Motivation Program
Pilot Projects
How to Pay for Decarbonization
Community Discussions
Workshops
Executive Summary
There are two primary means of harvesting solar energy, a free and carbon-free source of energy available across the planet: photovoltaic (PV) production of electricity and solar thermal systems which produce heat. Solar thermal may be used at utility scale to produce electricity, but the focus of this article is the direct and indirect use of solar energy as a source of space heat and domestic hot water.
Solar thermal collectors are 2-3 times more efficient at converting solar radiation to useful energy than PV systems. Thus, thermal collectors make better use of limited roof or site area, but thermal collector systems may be more costly to install, more complex, and require more maintenance than PV systems. All of these factors must be considered in evaluating solar thermal systems against alternatives.
Although humans have been using solar thermal energy in various ways throughout our existence, the systems that collect, store, and use thermal energy are not as packaged for commercial use as PV systems are, generally requiring more engineering, selection of components, and construction labor.
A distinct advantage over solar PV is that storage can be as simple as an insulated water tank. Hot water storage is less expensive, does not depend on lithium mining and trade, and can be more space-efficient (with >50 degF temperature difference) than lithium batteries.
Solar Thermal Water Heating
Technical Description
Design Considerations
Solar thermal systems absorb sunlight as heat, as opposed to photovoltaic systems, which convert solar energy into electricity. Solar thermal hot water (STHW) systems use a collector that transfers heat either directly to water or to an intermediate fluid, which then transfers heat to water. The indirect system may be used where there is a danger of freezing, so the heat transfer fluid is an antifreeze solution.
STHW can be used for domestic hot water (DHW) production, for heating hot water (HHW) production, or a combination of DHW and HHW.
The simplest form of STHW is a passive system, which leverages the density change of water as it heats, using buoyancy forces to cause circulation through a collector. Passive systems are typically smaller-scale, used for domestic hot water (DHW) production in residential or light-commercial applications. Because the scale of passive systems is generally not suited to hospitals, this section will focus primarily on active STHW systems.
Active STHW systems use pumps to circulate water or heat transfer fluid through collectors and either storage tanks (direct systems) or heat exchangers (indirect systems).
Storage tanks are used to buffer the temporal difference in capacity between source and load. With sufficient collector and storage capacity, there is potential to serve loads on a diurnal cycle, or even through multiple days of cloud cover. The storage medium, water, is itself inexpensive, but the cost and space requirements of insulated storage tanks may limit storage capacity.
Indirect systems utilize a heat transfer fluid that is protected from freezing under anticipated conditions. Aqueous solutions of propylene glycol or ethylene glycol with corrosion inhibitors are common. Ethylene glycol is toxic, whereas propylene glycol is “generally recognized as safe” for humans. The heat transfer fluid is used to transport energy from the collector to either DHW or HHW, via a heat exchanger.
There are many types of solar collector:
A simple flat plate collector may be constructed of black-painted tubing with a black background and a glass cover - the internet, and magazines before the internet existed, feature numerous designs for DIY flat plate collectors. Flat plate collectors are effective, though even commercial grade collectors are relatively inefficient and must either be filled wit antifreeze fluid or drained down in cold weather.
Evacuated tube collectors are commonly used in commercial applications. These collectors enclose a heat pipe within a glass tube and a vacuum is drawn within the glass tube. Without air in the tube, convective and conductive heat transfer is eliminated (mostly, since the vacuum is never perfect), but solar radiation passes through uninhibited. A heat pipe is a sealed metallic tube partially filled with a refrigerant. Pressure inside the tube is such that, as the tube is heated, the refrigerant boils, forming a hot gas that rises up the vertical or sloped tube. The top of the tube is pressed into a metal header, where water of heat transfer fluid is circulated. The gaseous refrigerant transfers heat to the circulating fluid, causing the refrigerant to condense, whereupon it flows down the sides of the tube to be reheated. Thus, a small direct expansion refrigeration cycle sets up within the heat pipe, driven by heat rather than a compressor. While this may sound complicated, the collector application is quite simple - all water or heat transfer fluid is contained in the hot part of the collector, at the top manifold, and - bonus - the refrigeration cycle does not reverse, limiting losses when solar radiation ebbs.
Another type of solar collector is the parabolic trough, which uses a curved mirror to concentrate solar radiation onto a tube that runs the length of the trough. Tube and trough run more or less level, with the trough mirror tilted along the longitudinal axis toward the sun. In some cases, the mirror rotates during the day to follow the sun. The tube is protected by an evacuated tube, but does not use a heat pipe like the evacuated tube collector, because heat pipes don’t work in the horizontal position. Because these are concentrating collectors, they are capable of higher temperatures, which are not usually needed for heating or domestic hot water, but they can be useful to drive absorption chillers or even generate steam.
Solar thermal collectors produce more usable energy per unit of surface area than photovoltaic panels - approximately 2-3 times as much. Thermal collector conversion efficiency is about When roof or siting space is at a premium and there is a use for thermal energy, STHW systems may be more effective at reducing greenhouse gas emissions than PV systems.
Thermal energy storage uses water or thermal fluid, requiring none of the rare metals and global trade of lithium ion batteries. Thermal storage is also more space-efficient when a temperature rise of at least 50 degF is maintained.
The major design drivers of STWH are:
- The amount of space available, typically but not exclusively on a roof
- Shading of the site
- Available solar radiation at the site, considering climate and latitude
- Storage capacity needed to balance supply and demand on the system.
Control strategies
Controls can be simple on/off depending on solar radiation or more complex strategies that include tracking systems and storage management. It is also essential to consider that collectors collect heat whenever the sun shines, whether there is load or not. There must be a system for managing excess heat to prevent high temperatures, high pressures, and unintended production of steam.
Solar tracking mechanisms add complexity and expense, but they maximize the energy gain of the system and reduce variability throughout the day - depending on the type of collector. Evacuated tube collectors “passively track” the sun through the day because the glass tube permits a better angle of incidence as the sun moves, compared to a flat plate, which has an optimized angle only once in the day. Consequently, evacuated tube collectors are typically installed in a fixed position.
Management of storage is essential for any system that must provide heat continuously. Without storage, STHW systems operate only while the sun shines and must be supplemented at night and during cloudy periods. Even with storage, a backup system will likely be needed for extended periods of cloud cover.
Best practices
Determine whether solar thermal is appropriate for your site based on insolation analysis. This will determine how much solar energy is incident on the location, throughout the year. Figure 1 below shows average daily solar radiation for the United States.
Figure 1. Average Incident Solar Radiation Received Per Day
For the majority of the continental United States, the total energy available is 4 kWh/m2/day (1.26 kBtu/sqft/day) or more. If the typical large hospital has a roof-to-floor area ratio of 5 (average of 5 stories), the average solar radiation on the roof is equivalent to 92 kBtu/sqft/year, or roughly 83% of the heating and DHW load of a large existing hospital. Solar thermal could be a significant part of the energy mix of a hospital, combined with load reduction, energy recovery, other sources, and thermal storage.
For heating and domestic hot water, both relatively low-temperature applications, fixed-position evacuated tube collectors are likely to be the best choice, due to higher efficiency than flat plate collectors, lower cost than concentrating parabolic troughs, and simplified piping.
How does this decarbonize?
Using solar-thermal water heating reduces or eliminates the need for fossil fuel boilers, electric heaters, and heat pump heaters for space heating and domestic hot water, replacing combustion or electric heat with renewable energy.
According to the 2012 CBECS data released by the US Energy Information, the average EUI of large hospitals was 234 kBtu/Sqft/Year and the average large hospital was 644,300 sqft. The analysis of Legacy Salmon Creek Hospital reported in Targeting 100! Showed that 44% of building energy consumption went to building heat and domestic hot water. Fossil gas releases 117 lb of CO2 per million Btus. So, the average annual CO2 emissions due to space heat and hot water in a large hospital is approximately 7.7 million pounds. STHW has potential to eliminate 100% of those emissions, though a more reasonable estimate is maybe 80%, or 6.2 million pounds of CO2e.
Implementation
Barriers: Technical
As with any solar installation, the system only works when solar radiation is available. Even with storage, there are limits to how long the system can function without additional energy input. Larger collector area and storage volume can increase ride-through time, but must be weighed against added cost.
There is potential to improve storage capacity using phase-change materials, which leverage the latent heat of phase change to store energy and maintain tighter temperature control than a stratified water tank.
STHW systems generally will not provide 100% of a hospital’s HHW or DHW, so a supplemental energy source is still required. The leads to either duplication of costs or economic optimization that results in less of the annual load carried by solar.
Barriers: Culture
Solar thermal systems have a reputation for being complex, unreliable, and prone to damage from freezing. For the most part, that reputation was earned by systems installed in the 1970s and 1980s, but still lingers. Experience from those systems as well as from a more active solar thermal market in Europe should be able to increase confidence, but engineers will need to see and read about more positive experiences.
The term “going solar” has largely come to be synonymous with solar PV. Economies of scale have greatly lowered the cost of production even as engineering innovation has increased the conversion efficiency of PV panels. Combining these improvements with relatively simple installation and very low maintenance, PV systems dominate the renewable energy market in the US. Solar thermal water heating is relatively unknown by the general public, even though it was in use for decades before the advent of PV. Government subsidies for solar development and manufacturing have been directed to PV, not thermal, adding to the perception mismatch.
Due to a smaller market share of solar projects, there are fewer suppliers of solar thermal water heaters and equipment.
Barriers: Financial
One useful metric for comparing energy systems is Levelized Cost of Energy (LCOE), which considers the cost of equipment and installation, maintenance, and energy produced over the life of the system. If energy is stated in similar units, this can be a useful comparison tool. 2020 estimates from the US DoE are that commercial PV installations have an average LCOE of $0.09 per kWh ($26.3 per MMBtu) and they project that the cost will approach $0.04/kWh ($11.72/MMBtu) by 2030. Although solar thermal is more space efficient and directly creates hot water, the resulting cost of energy can be near $13/Therm [KP Pilot Project], compared to about $1.76/Therm for PV [energy.gov]. This is a direct result of the current energy economic market. PV has enjoyed numerous government sponsored subsidies and large market interest, each driving down installed costs.
Strategy
- Determine energy loads for heating and DHW. Consider ways to reduce the heating load, so that investment in heating systems is minimized.
- Use hydronic heating systems for new construction and renovations. Hydronic heating systems allow flexibility in energy sources - electric, heat recovery, heat pumps, solar thermal.
- Consider site capacity for solar installation. The higher energy density of solar thermal may be an advantage on space-constrained sites.
- Consider all sources of thermal energy available, including heat recovery chillers, heat recovery from kitchen refrigeration and IT cooling systems, solar thermal, waste water heat recovery.
- Consider thermal energy storage to balance diurnal loads and/or seasonal loads. Seasonal loads may require large-scale storage, for which earth storage, such as borehole thermal energy storage, may be appropriate.
- Heat pumps are an effective way to transfer and lift energy from low-temperature sources and thermal storage to a usable temperature.
- Consider incentive programs, which may reduce the installation cost of some technologies, including solar thermal.
The optimal solution may be a combination of sources, storage, and heat pumps. Hourly annual load and source profiles will be necessary to determine the optimal mix of sources and storage. Consider extreme weather events as well, which will not be reflected in Typical Meterological Year (TMY) profiles.
Financial analysis and business case
Passive systems are cheaper and last longer, but are not as efficient as active systems. At a hospital in Jordan, a 1,133 sm had a payback period of 4.4 years, based off of a 700,000 USD investment. Pushes in funding renewable energy, there are some tax credits available for installation of solar thermal systems. There are also many opportunities for grants from private donors, and the Department of Energy, with an application. Financial savings can be determined by considering the pumping costs offset by the energy savings from avoiding usage of conventional boilers.
Case Study: Adams County Detention Facility
One large application is the Adams County Detention Facility in Colorado, which uses a parabolic trough system to produce 2 billion-Btus yearly from a 7,200 sf (less than 1/4 acre).
Case Study: Jordan Hospital
The largest public hospital in Jordan, Al Bashir, announced in 2018 that its design for a solar thermal hot water system had an estimated simple payback of about 4.5 year. The investment cost of the system was approximately $700,000 with an estimated annual energy savings of 1,000,000 kWh, and 176,000 liters of diesel fuel. Three heat exchangers linked the solar installation with the existing hot water supply system in the buildings. The heat exchangers were to provide preheating of steam boiler makeup water, heating hot water, and domestic hot water.
Comments
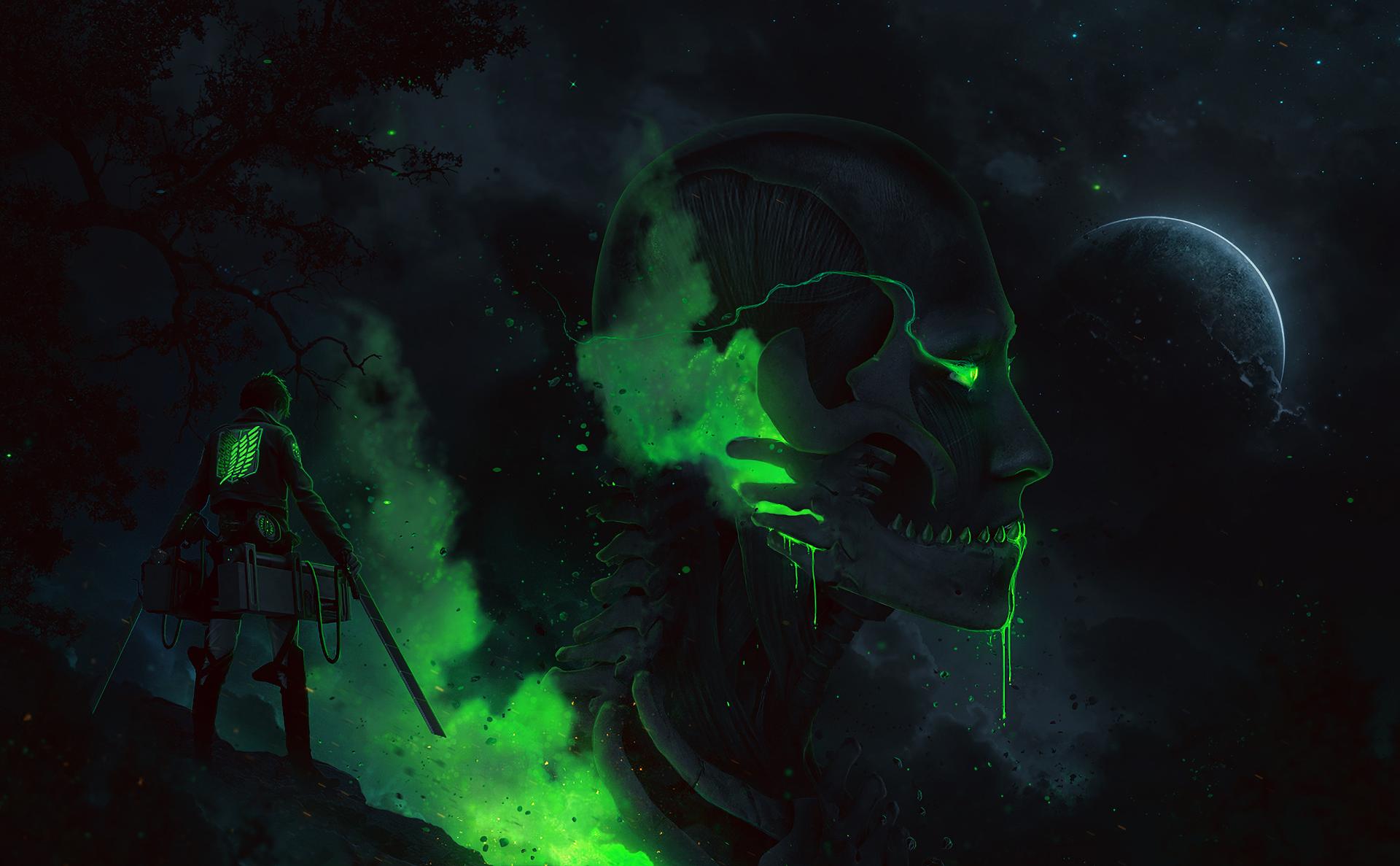
John Doe
8 seconds ago
Lorem ipsum dolor sit amet consectetur adipisicing elit. Quisquam, voluptatum. Lorem ipsum dolor sit amet consectetur adipisicing elit. Quisquam, voluptatum.
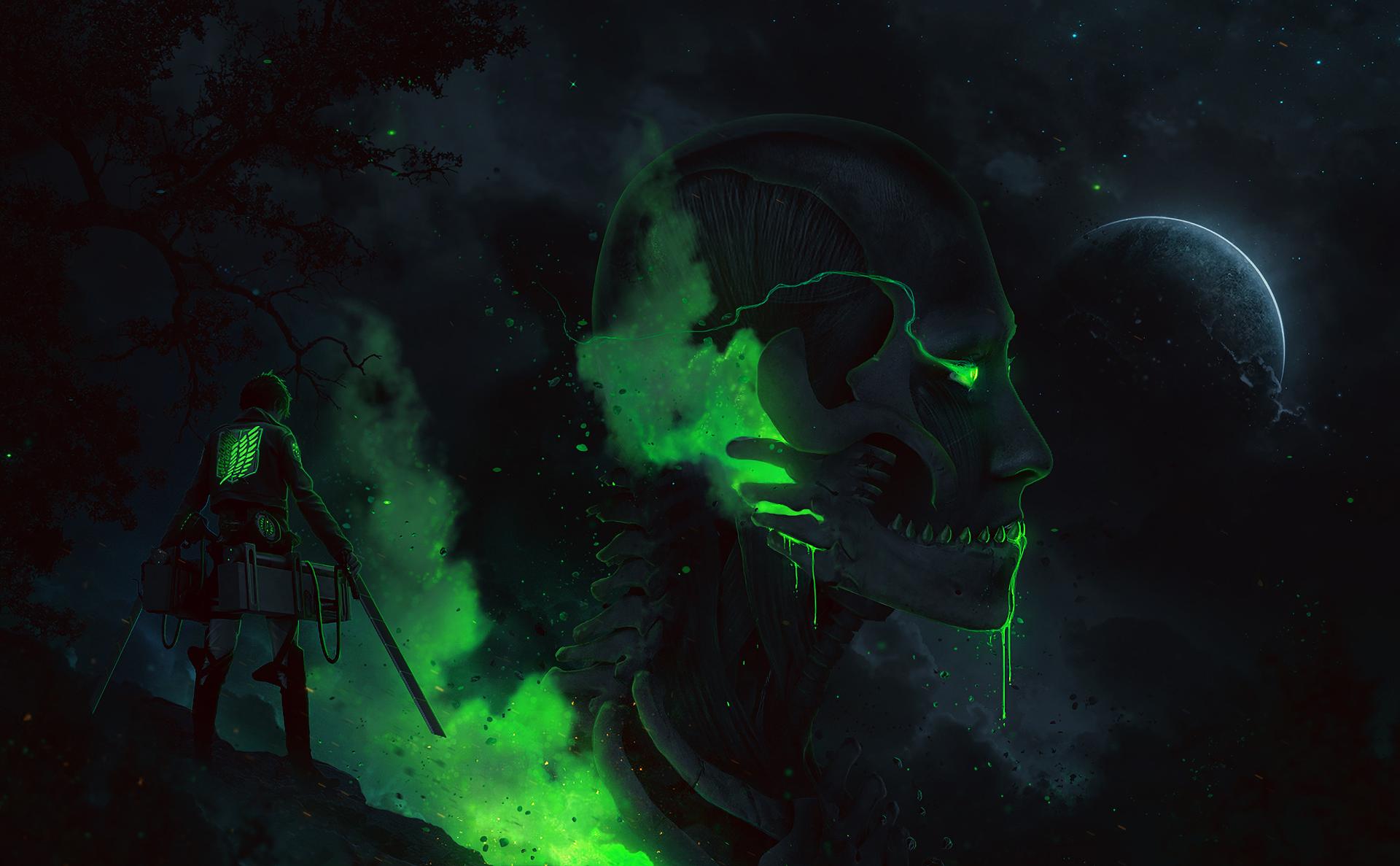
John Doe
18 seconds ago
Lorem ipsum dolor sit amet consectetur adipisicing elit. Quisquam, voluptatum. Lorem ipsum dolor sit amet consectetur adipisicing elit. Quisquam, voluptatum.