Welcome to the Decarb Guidebook
Approach
Benchmarking
Building Codes & Design Standards
State & Local Regulations
Technologies: Load Reduction
Technologies: Dehumidification
Technologies: If you must have a gas-fired boiler
Technologies: Central Plant
Technologies: Domestic Hot Water
Technologies: Steam
Technologies: Load Shifting and Energy Storage
Technologies: Emerging Technologies
Motivation Program
Pilot Projects
How to Pay for Decarbonization
Community Discussions
Workshops
Executive Summary
Newer onsite conversion technology (Waste-to-Energy) systems provide an opportunity to reduce carbon emissions associated with waste disposal. These systems reduce carbon emissions in two ways. First, waste (municipal waste, medical waste, etc.) is used to create thermal energy that can be used for heating domestic hot water. Second, carbon emissions associated with transportation of waste are eliminated and disposal associated emissions are reduced. Note, carbon emissions associated with transportation of waste are typically Scope 3 reductions and therefore are not inherently part of the calculation for systems considering. These systems could be sized and used for all hospital waste, however medical waste is likely the best opportunity. This is because medical waste is more expensive to dispose (strengthening the financial case) and facilities are typically further away (providing greater opportunity for waste transportation associated carbon reductions.) Importantly, these systems can typically be used for handling medical waste. They typically use a small quantity of gas for start-up, then run on energy produced from the waste.
Waste to Thermal Energy
Background
Hospital Biomedical Waste Disposal
Most hospitals dispose of biomedical waste in two ways: costly-off-site treatment of regulated medical waste through third-party services or on-site disinfection followed by off-site disposal of sterilized waste via third party services. The off-site treatment of biomedical waste using third-party waste haulers is the most common method of waste disposal in North America. In this scenario, the regulated medical waste is transported by a refrigerated truck to a contractor owned/operated waste management facility, where it is either incinerated or autoclaved and then landfilled at great financial and environmental cost. The transportation, incineration, and landfilling of medical waste is a contributor to greenhouse gas emissions, especially when medical waste is subjected to long-distance transportation. The second most common option is a combination of on-site and off-site treatment procedures. In some cases, the hospital opts or is required to disinfect the medical waste on-site before being transported to landfills or incineration for final treatment.
In the United States, after passage of the Medical Waste Tracking Act of 1988 (that required medical facilities to treat and track waste) many hospitals installed on-site incinerators to dispose of medical waste. These systems resulted in emissions that became a serious threat to environmental and human health. Of particular note, they were significant sources of dioxin and furan emissions. Most of the systems couldn’t meet clean air standards. Hospitals, for the most part, stopped using these incinerators, shutting them down completely. Onsite waste disposal (through incineration) grew out of favor because of this experience.
There are relatively few commercial incinerator operations in the United States. Since many jurisdictions require incineration of portions of the medical waste stream (specifically pathological and pharmaceutical waste) this necessitates waste being hauled long distances for treatment.
Onsite Waste to Energy (Conversion Technologies)
Newer Waste to Energy systems may offer an alternative to traditional medical waste management. Such systems convert almost any combustible material, including medical waste, into renewable thermal energy and bio-char - a charcoal like substance made from the decomposition of organic material. These systems typically are designed to recover energy contained in the by-products and use it as clean fuel for the process, delivering excess energy in the form of hot water to the site where it is located. The newer waste to energy systems are not incinerators but are instead based on Conversion Technologies. Conversion Technologies provide an opportunity to use biological, chemical and non-incineration thermal technologies to convert solid waste into clean energy (typically syngas.) Through the on-site treatment of medical waste, waste to energy systems can help hospitals reduce GHG emissions in addition to potentially reducing waste management costs. It is important to understand the emission profile of these systems. Most new on-site waste to energy systems have significantly reduced or eliminated dioxin and furan emissions and therefore do not raise the same environmental concerns as previous onsite medical incinerators. These incinerators are often referred to as “waste to energy” systems. Therefore, when considering such a system it is important to ensure the technology being used is not incineration.
Note, per the Greenhouse Gas Protocol, “Only waste treatment in facilities owned or operated by third parties is included in scope 3. Waste treatment at facilities owned or controlled by the reporting company is accounted for in scope 1 and scope 2. Treatment of waste generated in operations is categorized as an upstream scope 3 category because waste management services are purchased by the reporting company.” This means that offsite waste disposal is a Scope 3 emission. Emissions from onsite waste disposal may be considered scope 1. If an organization is only considering Scope 1 and Scope 2 emissions in a carbon reduction program, onsite waste to energy may not appear to reduce emissions, even though overall emissions (including scope 3) may significantly decrease.
The main carbon reduction benefit of these systems is reducing carbon emissions from the transportation and disposal of the medical waste stream. There is also ancillary reduction of scope 1 heat since the waste to energy system can provide heat for domestic or heating hot water.
Some of these conversion technology systems can be sized and placed on a skid such that they are practical for onsite use. They do require staff to be trained for operation.
Process Background
Onsite Conversion Technologies typically use pyrolysis or gasification to convert waste to energy. These conversion technologies are not combustion as they do not involve burning.
Gasification. In the gasification process, organic materials are converted to CO and H2 (syngas) by controlling the temperature and the amount of oxygen. A product of this process is bi-char.
“Gasification is a process in which organic waste is partially oxidized to form chemical reactions to produce carbon dioxide, carbon monoxide, and methane gases that create extreme high temperatures in the gasifier. The syngas that is generated from the three primary gases can be utilized for industrial and commercial process and products, including photographic film, coal, and petroleum, while the solid residue (slag), made non-hazardous by cooling, can be used for a variety of manufacturing products. Some versions of this technology use the gas to fuel the process, and extract heat energy from the system for use as an energy source.” Detailed information on gasification is available from the National Energy Technology Laboratory (https://netl.doe.gov/research/Coal/energy-systems/gasification/gasifipedia/intro-to-gasification).
Figure 1. Gasification Process Basics Breault [1]
Pyrolisis
Pyrolysis is an oxygen-free thermal treatment process used to decompose organic materials. Pyrolysis produces solid (biochar) liquid and syngas (non condensable gasses.) Waste is typical processed between 750
“Pyrolysis is an oxygen-free thermal treatment process that processes waste at temperatures between 750ºF and 1500ºF in the absence of air.[1] The lack of oxygenation is a critical difference between pyrolysis and combustion. The fuel used to initiate the “baking” of the waste can be natural gas, propane, or the gas generated by the pyrolysis process itself. This process first uses a pyrolytic chamber that reduces the waste to ashes and gases, and uses a “post-combustion” chamber to burn the produced gases at very high temperatures. This resulting synthetic gaseous (syngas) product of this and other conversion technologies is often referred to as syngas, and consists of hydrogen, carbon monoxide, and methane.”
Waste to Energy
Waste-to-energy systems built off these conversion technologies simply take the syngas produced and use it for beneficial use. Typically these systems require a small amount of energy (from natural gas or propane) to startup and then use the gas produced to continue operations. [2]
Residual Disposal
Creating bio-char reduces the amount of CO2 in the atmosphere because a portion of the carbon in the waste material is recovered in the bio-char. While in some applications, the bio-char can serve as a soil amendment to enhance nutrient and water retention, thereby potentially reducing water and fertilizer consumption, for a system that is processing medical waste such as is suggested herein, the biochar would need to be landfilled. These systems send approximately 5% of input (by mass) to landfill
Technical Description
Design Considerations
Design considerations are based on the specific waste-to-energy manufacturer/system selected. The following are stylized consideration for a waste-to-energy system
Size
For an on-site pyrolysis or gasification based system it's advisable to use a relatively small, modular system. This is true especially for existing buildings that may want to add such a system at the loading dock or other location where waste is processed today. Such a system may have the following approximate characteristics:
- Size: 11 ft x 8 ft
- Height: 2ft
- Weight: 6 tons
- Processing rate: 40 - 80 tons per hour. Rate is dependent on waste stream composition
- Energy production: For a unit this size say ~120kW when operating at full capacity
Output
The output of a modular onsite unit would produce heat that might be used in a heating or domestic hot water system. It would not be expected to produce heat for a steam system.
Location
It is typically assumed that a hospital would locate their unit(s) in or near the loading dock since this is where waste is typically processed and loaded today. There will also need to be consideration given to tying the system into the hospital hot water system or whatever system will use the energy produced. Careful consideration should be given depending on the particular site.
Best Practices
Once an organization sees the potential for an onsite conversion technology system (waste to energy) an analysis should be performed to determine the potential impacts on energy use and the potential reduction in waste stream carbon emissions. To implement this strategy, the engineer will want to perform a detailed analysis including a full understanding of waste volumes (especially medical waste volumes) and associated carbon emissions for their waste stream. Understanding current waste hauling distances and associated emissions factors is important.
The size of facility, volume of waste produced and space considerations all have implications for how an onsite waste to energy system would be operated. Therefore, there are several factors to consider in choosing a waste to energy system and, assuming a modular unit, how many units to use. These conditions include:
Unit run time:
- Days per week of operations
- Weeks per year of operation
- Hours per day
- Number of units
Staffing
- The above provides consideration of staffing.
For one unit, a staff person will need to be there, but will likely not spend all of their time operating the waste-to-energy unit. For estimating purposes it can be assumed that 1 full time employee (FTE) can operate 2 units. If only 1 unit is operated assume 60% of a person's time would be dedicated to unit operation. This includes starting up the machine each day, feeding waste into the machine and general operation and maintenance.
How does this decarbonize?
Generation of energy on-site from waste reduces consumption of energy from other sources, thus decreasing the environmental footprint and cost of producing and transporting that energy to the hospital. Through onsite conversion of medical waste to energy, the Hospital Partner will likely create a net decrease in pollutants and greenhouse gasses emitted due to the elimination of long-distance waste transportation, incineration/autoclaving without energy recovery. Additionally, onsite units are estimated to reduce waste to landfill by 95%. Further, because waste is mostly managed onsite, the residual risk of off-site spilling, contamination, and pathogen introduction is vastly reduced.
Implementation
Barriers: History
Implementation of onsite waste to energy systems may be hampered by the general history of waste to energy system and by existing waste disposal methods. Onsite incineration systems developed a poor reputation in US healthcare because of their emission profiles (high furan and dioxin emissions). Newer systems do not have the same emission profile but there may still be hesitance to adopt these newer systems because of that history.
Barriers: Culture
Waste hauling organizations are incentivized to continue hauling waste and have made investments in growing their fleet of trucks. Onsite waste-to-energy systems reduce their revenue. The waste-to-energy systems are most environmentally and financially advantageous for medical waste, however hospitals generate other waste streams that may still need to be hauled. Thus, relationships and setup of hospitals with existing waste haulers might be a barrier to implementation since these relationships may be complex and there may be financial, logistical or other reasons to maintain the status quo.
Barriers: Finance
Installing a waste-to-energy system requires capital to purchase the system or have an alternative service model. Depending on the setup, hospitals may have to break contract with current waste hauler.
At the moment, the financial payback on these systems are likely very small or zero. Site level analysis will need to be performed, but these systems may not provide a great financial return on investment.
Even in cases where the onsite waste-to-energy system does not provide a great financial return on investment, it will provide a return from reduced environmental harms and solves a major problem.
These benefits and costs may hit different budget lines and therefore be more difficult to implement. Detailed cost estimates will need to be performed. Depending on labor costs, energy costs, etc. the impacts to net margin will vary. In some cases there may not be an improvement.
Strategy
A strategy for a hospital to obtain a waste-to-energy system might involve first performing a pilot. Ideally the hospital would be eligible for government grants or other funding to pay for the pilot.
The pilot may help derisk getting regulatory approval for the waste-to-energy system. Since these systems have not been used onsite in healthcare, derisking the approval process is important. And, this would potentially (depending on the funding setup) significantly reduce the capital costs for the system, since these costs could be paid during the pilot.
Once approval is obtained, continue to operate the now-in-place MAGS system.
Opportunities
There is the possibility of a company offering waste to energy as a service. The service would offer hospitals to treat their on-site waste at a specific rate ($/lb) of waste processed. The advantage of this approach is that a hospital would not have to pay the upfront equipment cost. Such a model may work particularly well in a location with several hospitals in close proximity who might be able to share cost and significantly reduce transportation distances.
Case Study: MAGS
Micro Auto Gasification System (MAGSTM) is a small onsite conversion technology being used on board various types of cruise ships, commercial ships and in several remote communities (https://terragon.net/applications/). MAGS is based on gasification with a gasifier operating at 1100F and the combustion chamber operating at 2000F. It uses about 4.5 gallons of fuel (natural gas, or an oil product) for heat up and also uses 60ml/Kg solid waste of NaOH, caustic caustic soda solution. MAGS is not currently installed in a hospital setting due primarily to hurdles associated with implanting new innovative technologies and environmental challenges. Mazzetti and Terragon are working to implement pilot projects with healthcare facilities that are seriously looking at reducing/eliminating their carbon footprints in the coming years. In the case of a pilot project, Terragon and Mazzetti would provide the Hospital Partner with a Carbon Emissions analysis comparison with their business-as-usual waste management model at the end of the pilot project to demonstrate the results of implementing a waste-to-energy system.
Comments
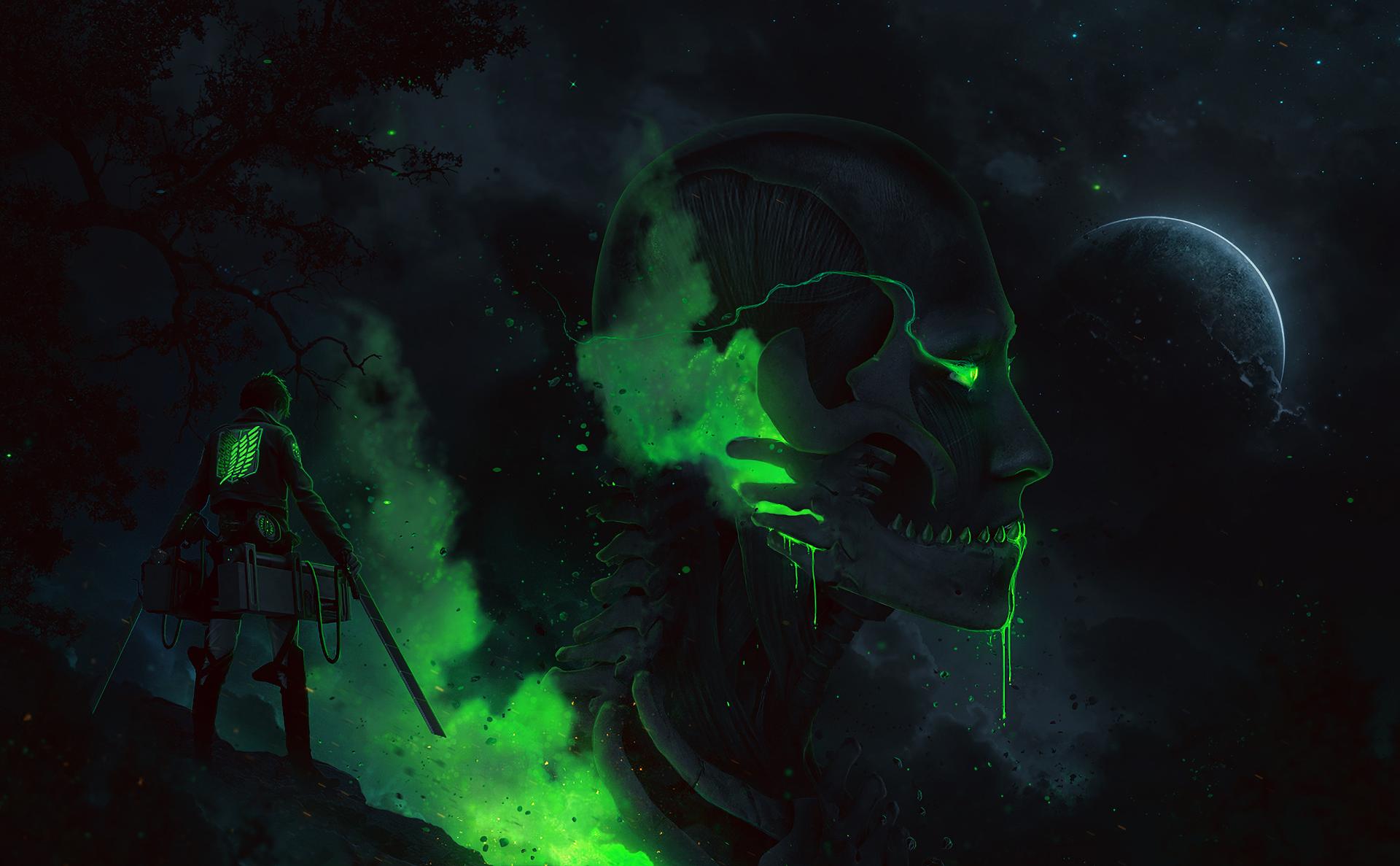
John Doe
8 seconds ago
Lorem ipsum dolor sit amet consectetur adipisicing elit. Quisquam, voluptatum. Lorem ipsum dolor sit amet consectetur adipisicing elit. Quisquam, voluptatum.
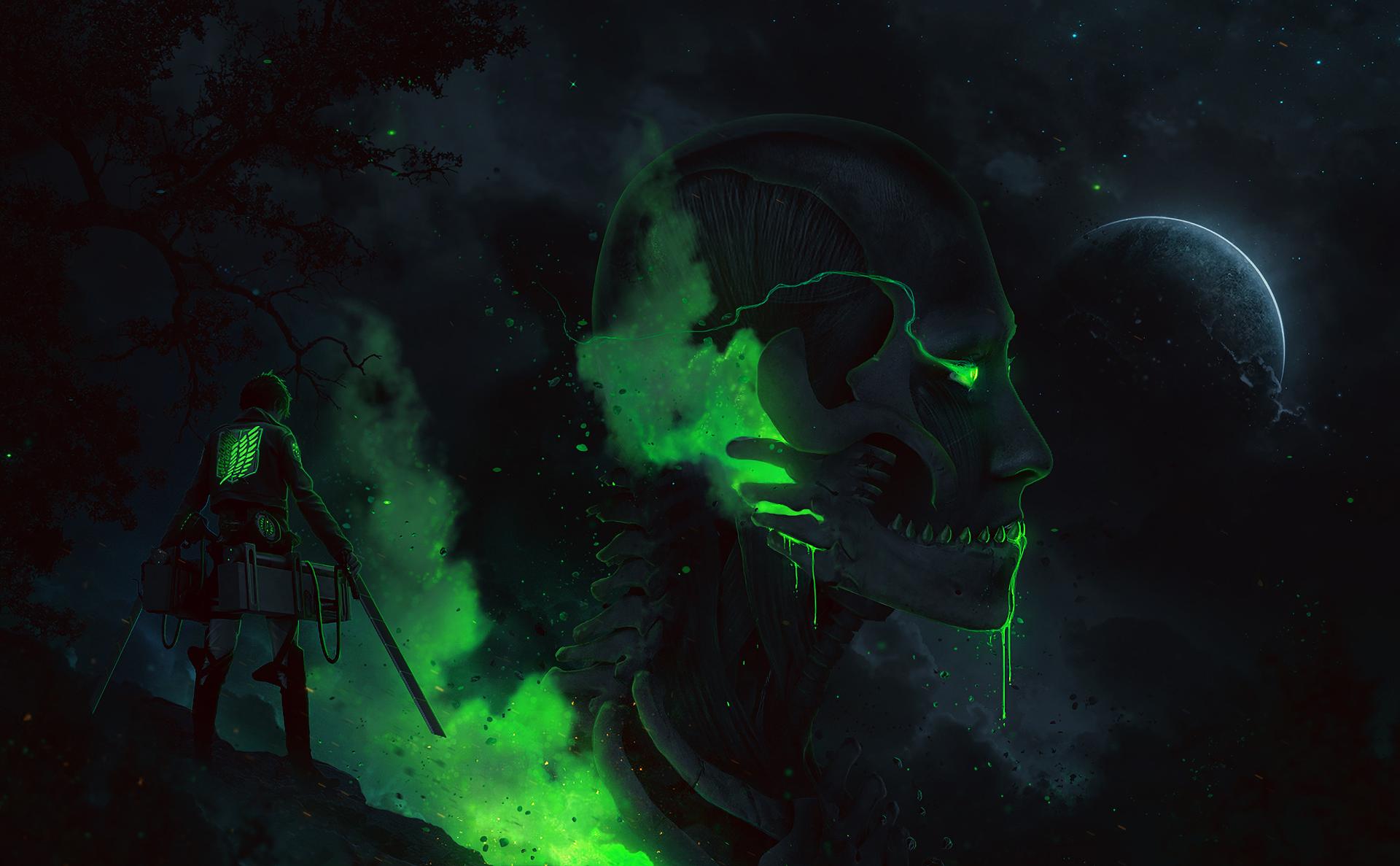
John Doe
18 seconds ago
Lorem ipsum dolor sit amet consectetur adipisicing elit. Quisquam, voluptatum. Lorem ipsum dolor sit amet consectetur adipisicing elit. Quisquam, voluptatum.