Welcome to the Decarb Guidebook
Approach
Benchmarking
Building Codes & Design Standards
State & Local Regulations
Technologies: Load Reduction
Technologies: Dehumidification
Technologies: If you must have a gas-fired boiler
Technologies: Central Plant
Technologies: Domestic Hot Water
Technologies: Steam
Technologies: Load Shifting and Energy Storage
Technologies: Emerging Technologies
Motivation Program
Pilot Projects
How to Pay for Decarbonization
Community Discussions
Workshops
Local heating systems provide a decentralized approach to building heating design. These systems which are much smaller than a traditional main central utility plant, offer more flexibility in heat generating technologies such as solar thermal, electric boilers, and heat pumps. This system can improve efficiency and reliability, as well as reduce operating costs and carbon emissions.
Executive Summary
Local heating systems have several benefits over central plant systems when it comes to decarbonizing a hospital. Firstly, local heating systems can be more energy-efficient than central plant systems. They deliver heat directly to individual buildings or units, reducing transmission losses and waste heat compared to a central plant system. Secondly, local heating systems can have a lower carbon footprint than central plant systems due to the use of all-electric heating, especially when coupled with on-site renewable energy such as solar, wind, or geothermal, which emit no greenhouse gasses. In contrast, central plant systems typically rely on large-scale fossil fuel combustion, which can result in higher carbon emissions.
Local heating systems can also offer more flexibility than central plant systems. Hospitals have different heating and cooling needs in different parts of the building, depending on factors such as occupancy levels and equipment requirements. Local heating systems can be customized to meet the specific needs of individual buildings or units, ensuring that the heating and cooling requirements are met efficiently. In contrast, central plant systems are typically designed for large-scale heating and cooling applications, which may not be suitable for all parts of a hospital building.
Local Heating Systems
Technical Description
Background
Local heating systems have been around for centuries and have been used in many different forms, including wood-burning stoves and geothermal heating systems. These systems have evolved over time, and today, they can be powered by a range of energy sources, including electricity, natural gas, and renewable sources such as solar and geothermal energy.
Central plant systems, on the other hand, were developed in the 19th century and have been widely used in large commercial and institutional buildings. These systems typically consist of a central heating plant that generates heat by burning fossil fuels to produce hot water or steam, which is then distributed through a network of pipes to individual buildings or units. A common central plant system in a institutional campus is a cogeneration system or combined heat and power system. Cogeneration plants involve the simultaneous generation of electricity and useful heat from a single fuel source. Typically, a cogeneration plant uses a gas turbine or internal combustion engine to generate electricity, and the waste heat from this process is captured and used for heating or cooling purposes. By using the waste heat, cogeneration can achieve overall energy efficiencies of up to 80%, compared to around 35% for a typical power plant that does not use waste heat. While cogeneration is more carbon-friendly than traditional power generation methods, it still produces greenhouse gas emissions due to on-site combustion.
In the context of decarbonizing buildings and reducing greenhouse gas emissions, there has been a growing interest in local heating systems due to the significant improvements in technology and efficiency such as heat recovery chillers, heat pumps, variable refrigeration system (VRFs) and smart controls to deliver heat more efficiently.
The choice between local heating systems and central plant systems often depends on factors such as the size and layout of the building, the availability of energy sources, the carbon emission condition of the existing central plant and whether there is a long term plan to upgrade the central plant to be more carbon friendly. It is important to conduct a detailed analysis of these factors before making a decision on which system to adopt.
Design considerations
When designing a local heating system, several factors need to be considered to ensure that the system is efficient, reliable, and environmentally sustainable. Here are some design considerations for a local heating system:
System size: The size of the heating system should be determined based on the heating load of the building. A detailed load model or energy model needs to be created to derive the optimized size of the heating system to ensure maximum system efficiency.
Fuel source: The choice of fuel source will have a significant impact on the carbon emissions and cost of operating the heating system.
Options for local heating systems:
Condensing Boilers: This system is covered in another chapter in the guidebook, please refer to Condensing Boilers for more detailed information.
Variable Refrigerant Flow (VRF): VRF is a type of HVAC (Heating, Ventilation, and Air Conditioning) system that provides both heating and cooling to a building. VRF systems work by circulating refrigerant between an outdoor unit and multiple indoor units. The refrigerant absorbs heat from the indoor air when cooling is needed and releases heat to the indoor air when heating is needed. The amount of refrigerant flowing to each indoor unit is variable and can be adjusted to meet the specific cooling or heating needs of each room or zone.
Another advantage of VRF systems is their flexibility in design and installation. They can be customized to fit the specific needs and layout of a building, making them ideal for retrofits or new construction. VRF systems can also be designed to connect to a building's automation system, allowing for remote control and monitoring of the HVAC system.
Overall, VRF systems are a highly efficient and flexible option for providing heating and cooling to a building. However, they can be more expensive to install than traditional HVAC systems and require specialized maintenance and service.
Heat Recovery Chillers and Heat Pumps: This system is covered in another chapter in the guidebook, please refer to Heat pumps and Heat Recovery Chillers for more detailed information.
Smaller Air Source Heat Pumps: A smaller air source heat pump (ASHP) is a type of heating and cooling system designed for small commercial applications. It uses the outdoor air as a heat source in winter and a heat sink in summer to provide energy-efficient space heating and cooling.
A smaller air source heat pump can be a suitable option for heating and cooling smaller spaces in a hospital, such as individual patient rooms, offices, or smaller treatment areas.
Advantages of smaller ASHP include high efficiency, cost savings, environmental friendly and easy installation and maintenance. When considering a smaller air source heat pump, it is essential to select the right size and capacity for your specific needs.
Other Considerations:
Refrigerant: While heat pumps are a great alternative to heating systems that run on fossil fuel, they do rely on refrigerant to operate. Some of the traditional refrigerants like R410a have a global warming potential (GWP) more than 2,000 times that of CO2. Low GWP refrigerants, on the other hand, have been developed as alternatives to traditional refrigerants. Examples of low GWP refrigerants include hydrofluorocarbons (HFCs), hydrofluoro-olefins (HFOs), and natural refrigerants such as carbon dioxide, ammonia, and hydrocarbons. Selecting proper heat pump technology with low GWP refrigerant or natural refrigerant can be critical to achieving building decarbonization goals.
Efficiency: The efficiency of the heating system is important to minimize energy consumption and operating costs. High-efficiency boilers or furnaces, heat pumps, VRFs and radiant heating systems can be used to increase the efficiency of the system.
Distribution system: The distribution system carries heated air or water from the heating source to the building's interior spaces. It is important to design an efficient distribution system that minimizes heat loss and ensures consistent heating throughout the building.
Controls: Advanced controls can be used to optimize the performance of the heating system, including temperature sensors, thermostats, and schedule based or occupancy based controls.
Maintenance: Regular maintenance is essential to ensure that the heating system operates reliably and efficiently. This includes regular inspections, cleaning, and replacement of worn components.
Cost: The cost of installing and operating the heating system should be considered to ensure that the system is affordable and cost-effective over its lifetime.
By carefully considering these design considerations, local heating systems can be designed to be efficient, reliable, and sustainable, helping to reduce carbon emissions and minimize operating costs
Best practices
Here are some best practices for designing, installing, and operating a local heating system:
Choose the most efficient and cleanest fuel source: The choice of fuel source will have a significant impact on the carbon emissions and cost of operating the heating system. Renewable energy sources like solar, geothermal, and biomass can be used to reduce the carbon emissions of local heating systems.
Properly size the system: The size of the heating system should be determined based on the heating load of the building, which depends on factors like the size of the building, insulation, and climate. Proper sizing can help to minimize energy waste and optimize system performance. This is extremely critical for a heat pump system.
Use high-efficiency equipment: High-efficiency boilers, heat pumps, and radiant heating systems can be used to increase the efficiency of the heating system and reduce energy consumption.
Monitor energy consumption: Monitor the energy consumption of the heating system to identify opportunities for improvement and optimize performance. Use energy monitoring tools to track energy consumption and identify areas for improvement.
By following these best practices, local heating systems can be designed and operated to be efficient, reliable, and sustainable, helping to reduce carbon emissions and minimize operating costs.
How does this decarbonize?
As mentioned above, most of the central plant today uses fossil fuels like natural gas, coal, or oil to generate heat, which will emit carbon dioxide (CO2) and other greenhouse gasses into the atmosphere as a byproduct of the combustion process. The carbon emissions from a central heating plant can be calculated based on the amount of fuel used and the carbon intensity of the fuel. According to the US Environmental Protection Agency (EPA), the average carbon dioxide emissions from a natural gas-fired boiler used for heating purposes is approximately 117 pounds of CO2 per million British thermal units (lb CO2/MMBtu). This figure can vary depending on the specific technology and efficiency of the heating plant.
Local heating systems on the other hand, can be more flexible in choosing what source it uses. Traditionally, local heating systems have been relying on natural gas based boilers to provide hot water for building heating. With advanced boiler technology like condensing boilers, the system efficiency could be as high as 95% with low return water temperature. (For more details please refer to the condensing boiler chapter in this guideline). As high as the boiler efficiency could be, it still requires on-site combustion and emits significant amounts of CO2 and other greenhouse gasses.
As an alternative, electricity-based local heating systems can yield much lower carbon emission with heat pump technology. This is because the CA electricity grid is getting cleaner and is projected to be 100% carbon-free by 2045 required according to the state's Renewable Portfolio Standard (RPS). On-site renewable energy like solar and wind could also be used to offset the electricity based heating system, which do not emit greenhouse gasses.
Table1 below
Central Cogen Plant | Onsite Condensing Boiler | Local Heat Pump System | |
Natural gas Consumption (mmbtu) | 50,000 | 35,556 |
|
Electricity Consumption (mmbtu) |
|
| 10,667 |
Carbon emission (tons of CO2) | 2,925 | 2,080 | 619 |
Percentage of savings | N/A | 29% | 79% |
Table 1: Represents the carbon emissions saving potential of an on-site condensing boiler and a heat pump based local heating system compared to a non-condensing hot water boiler central plant system. This shows that the local condensing boiler system could save 29% in carbon emissions and the local heat pump system could save 79% in carbon emissions compared to the hot water boiler based centralized system.
Assumptions | Assumed Value |
Building heating demand annually (kbtu) | 32,000 |
Central plant heating efficiency | 80% |
central plant distribution efficiency | 80% |
on-site condensing boiler efficiency | 90% |
Heat pump annual efficiency | 3% |
natural gas carbon emission factor (lb/mmbtu) | 117 |
CA grid emission factor (lb/mwh) | 396 |
Table 2: lists the assumptions that go into the calculations for table 1
Implementation
Barriers: Codes
Air-to-air heat pump or water-to-air heat pump based system like air source VRF or water source VRF would need to be coupled with a dedicated outdoor air system (DOAS) to provide ventilation to the building to ensure health and safety requirements by the Department of Health Care Access and Information (HCAI). Table 4-A under chapter 4 of the California Mechanical Code dictates the ventilation requirement based on different space types. Because of this, most of the hospital space types like patient rooms, operating rooms, treatment rooms, etc. will be ventilation driven spaces in terms of the peak design airflow. This means that the DOAS system size would be as big as the traditional variable air volume (VAV) system and yield no cost savings. Therefore, the local VRF heating system is totally added cost and could be cost prohibitive.
The air-to-water heat pump or water-to-water heat pump does not have the same problem as it is generating hot water to serve the reheat coil in the VAV+reheat system. This type of design has gained some popularity recently due to the decarbonization requirement to electrifying heating systems. Some local jurisdictions have banned natural gas use for new construction for certain building types; even though institutional facilities like healthcare buildings are exempt from this requirement, the energy code is certainly encouraging localized heat pump systems.
Barriers: Culture
People are generally hesitant to adopt a new heating system if they are accustomed to using traditional methods. They may be skeptical about the reliability and effectiveness of a new system, and may be unwilling to invest in the necessary changes.
Local heating systems, in particular local heat pumps and VRF systems, are relatively new and a lot of engineers still need experience to understand how they work, or how to properly maintain them, which can ultimately undermine the effectiveness of the system.
In addition, some facilities may have specific preferences and requirements for heating systems based on their traditional practices and beliefs, which makes adopting new concepts of local heating systems challenging.
Barriers: Technology
The first heat pump systems were developed in the 1940s and 1950s and VRF technology was first introduced by Daikin Industries, a Japanese air conditioning manufacturer, in 1982. So they are not new technology. However, they are still relatively new in terms of implementing them to deal with the heating load for large buildings like a hospital. Technology barriers might include:
- System complexity: Heat pump systems can be more complex than traditional heating systems, with multiple components and controls that require specialized knowledge to install and maintain.
- Heat pump systems may require modifications to the building's electrical and plumbing systems, and may be more difficult to install in older buildings that were not designed with heat pump systems in mind.
- Technical expertise: Installing and maintaining a heat pump system requires specialized knowledge and expertise. This can be a challenge in areas where there is a shortage of qualified technicians, or where training and certification programs are not widely available.
Strategy
Established energy providers, such as utility companies, may resist the implementation of a local heating system, particularly if it threatens their market share or revenue streams. This can create regulatory and legal barriers to implementation.
Implementing a local heating system can be complex, and may require specialized technical expertise, as well as coordination with multiple stakeholders, including building owners, contractors, and local utilities.
To implement the local heating system, it is recommended to evaluate the existing heating hot water requirement, evaluate the trended data if available to get an accurate estimate on the heating demands. This would help avoid oversizing the heat pump system, which could be problematic to operate.
Financial analysis and business case
Implementing a local heating system can have both financial and business benefits, some of which are outlined below:
Financial Benefits:
- Reduced Energy Costs: By implementing a local heating system, you can reduce your energy costs by using more efficient and cost-effective heating sources, which can lead to significant savings on your energy bills.
- Increased Property Value: Installing a local heating system can increase the value of your property, especially if the system is energy-efficient and sustainable. This can be a selling point for potential buyers and help to justify the initial investment.
- Access to Incentives: In California, there are utility incentives available for hospitals to install energy-efficient and sustainable local heating systems. These incentives can help to offset the initial investment costs and provide long-term financial benefits.
Business Benefits:
- Reduced Carbon Footprint: Implementing a local heating system can help businesses to reduce their carbon footprint, which is becoming increasingly important for both regulatory and public relations reasons. This can help businesses to demonstrate their commitment to sustainability and environmental responsibility while providing flexibility over their heating needs.
- Increased Control and Flexibility: Local heating systems offer businesses greater control and flexibility over their heating needs. This can be particularly beneficial for businesses that have varying heating requirements, such as those with seasonal fluctuations or different operating hours.
- Enhanced Reputation: Businesses that implement local heating systems can enhance their reputation and brand image by demonstrating their commitment to sustainability and the environment. This can be an important factor in attracting customers and employees, as well as building a positive reputation within the community.
Overall, there are numerous financial and business benefits to implementing a local heating system. While there may be upfront investment costs involved, the long-term benefits can far outweigh these costs, making it a worthwhile investment for many businesses.
Case Study: Confidential CA Medical Center
A new hospital being designed and built in California will have approximately 955,000 square feet of new acute care space. It will have 384 beds in the new hospital for a total of 675 beds, 40 Operating Rooms and new Pre-Operative and Post-Anesthesia Care Unit support and 17 state-of-the-art interventional rooms. This project will be the centerpiece of a comprehensive plan to transform an entire campus that continues to drive innovations in care delivery, discovery and health sciences education and honors the University’s longstanding commitment to the community.
Instead of connecting to the existing campus cogeneration central plant, the project is planning to have a localized all-electric heat-recovery plant with two 600-ton heat recovery chillers as the primary heat source. Energy modeling results show that the proposed local heat recovery system will be able to reduce 30% of the carbon emissions compared with connecting to the existing centralized cogeneration plant.
Case Study: UC Davis California Tower
UC Davis California Tower
UC Davis California tower is an 850,000-gross-square-foot Hospital Bed Replacement Tower project at University of California Davis campus. The project will provide a state-of-the-art hospital facility with both intensive care unit and medical surgical patient beds as well as an interventional platform that supports new surgical techniques and technologies. The project is set for groundbreaking in 2023 and open to patients in 2030. The project is shooting for LEED Gold under LEED V4 healthcare.
A local heat recovery chiller/heat pump system to cover the base cooling load of the building was evaluated in the early design phase of the project and has proven to be super effective in both reducing the operating cost of the building as well as reducing the carbon emission of the building.
Figure 1. represents the carbon emission delta; a 165-ton HRC can reduce the overall building carbon emission by 6%
Comments
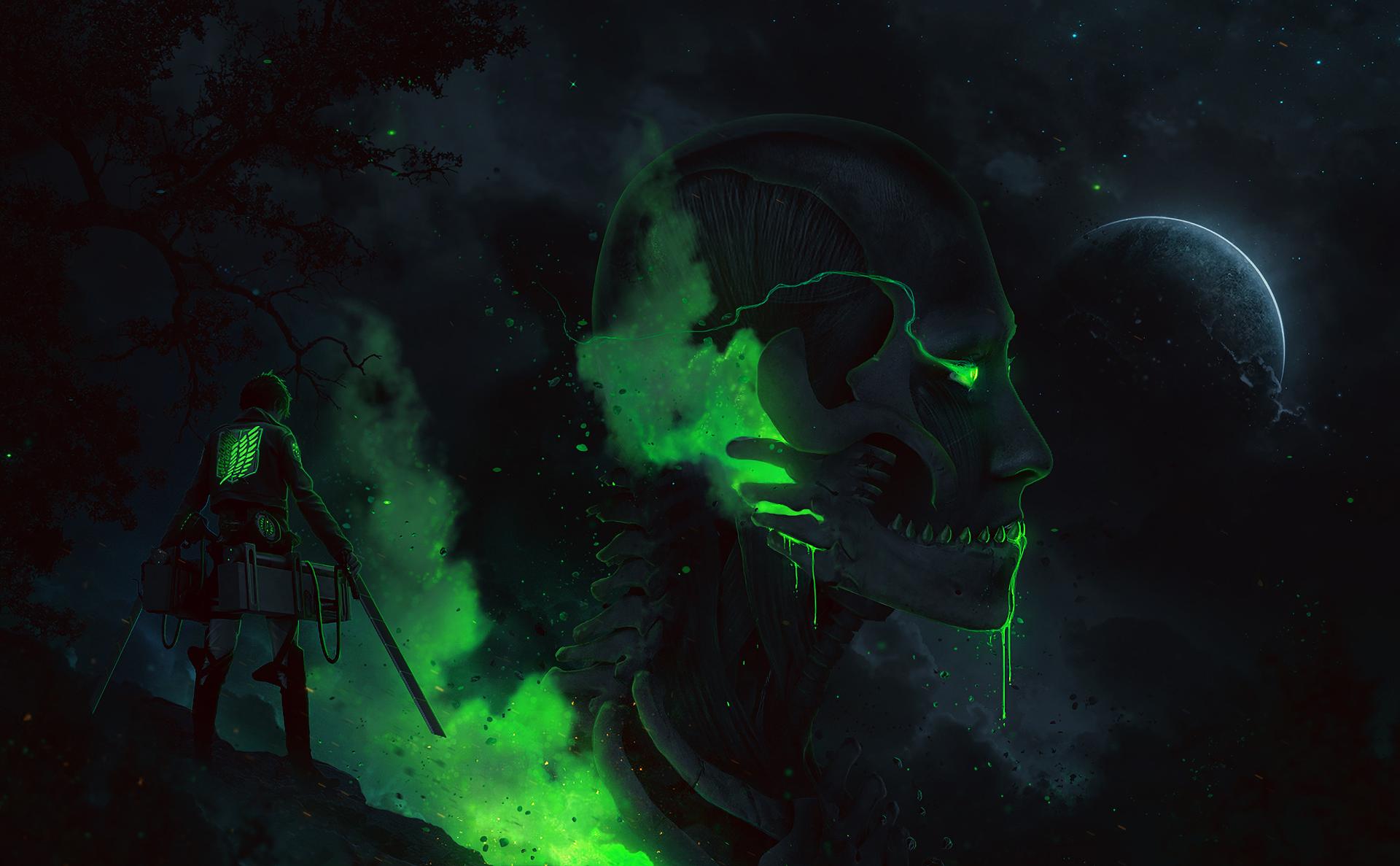
John Doe
8 seconds ago
Lorem ipsum dolor sit amet consectetur adipisicing elit. Quisquam, voluptatum. Lorem ipsum dolor sit amet consectetur adipisicing elit. Quisquam, voluptatum.
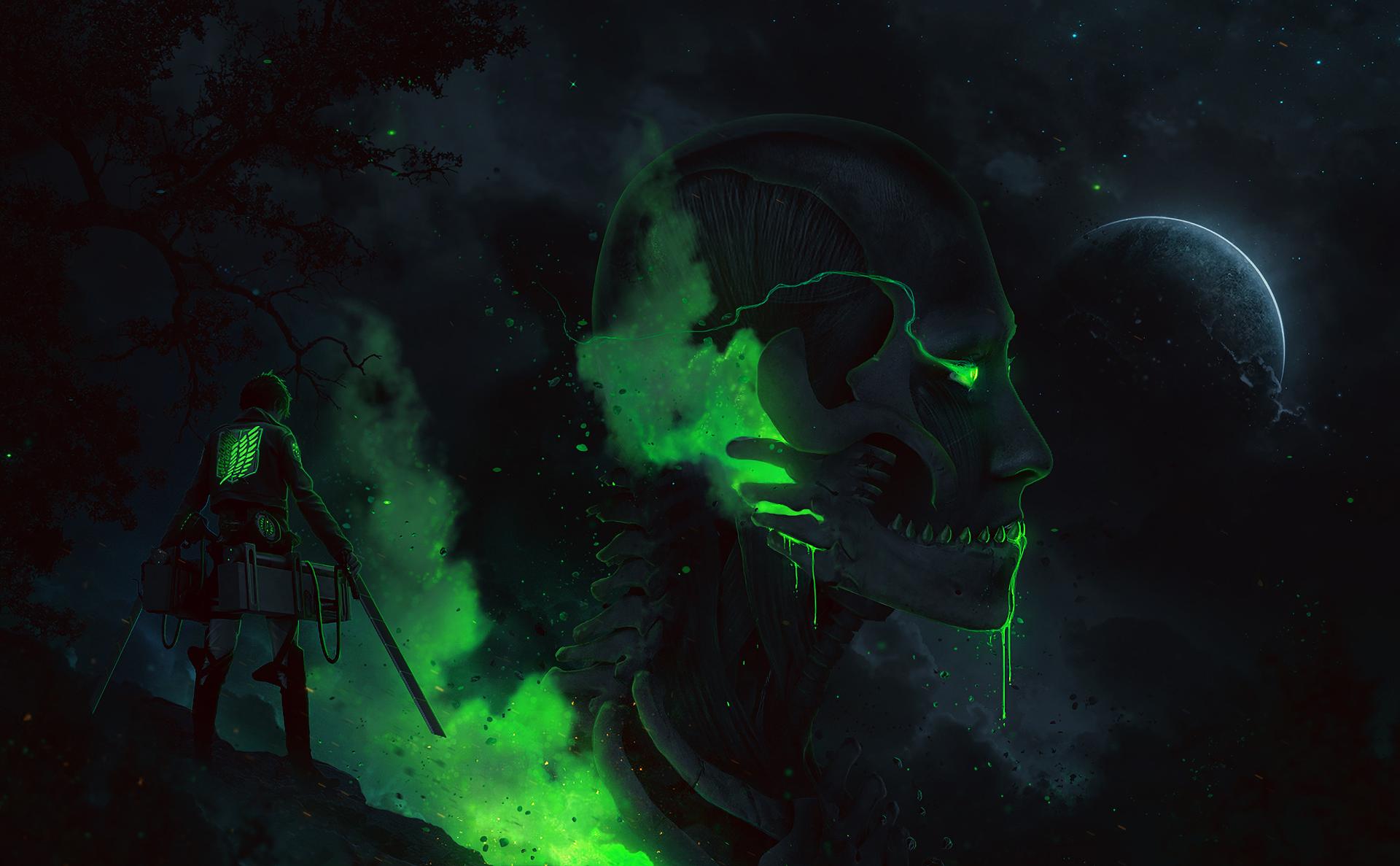
John Doe
18 seconds ago
Lorem ipsum dolor sit amet consectetur adipisicing elit. Quisquam, voluptatum. Lorem ipsum dolor sit amet consectetur adipisicing elit. Quisquam, voluptatum.